Behavior of Trace Elements in the Gaseous Phase during Combustion of Coal and Coal Waste
Behavior of Trace Elements in the Gaseous Phase during Combustion of Coal and Coal Waste
Abstract
The present study shows:
1) the results of field studies of trace element transfer from coal waste fires in different regions of Russia;
2) the results of laboratory experiments on coal combustion;
3) the results of field studies of trace elements in snow cover near coal-fired thermoelectric power stations (TPSs).
Comparison of the chemical composition of all three objects has shown that the character of the elemental distribution is similar in the dissolved part of the snow cover near TPS, in the vapour-gas condensates sampled on burning coal dumps and in the condensates obtained by the experiment. In the preliminary experiment, the condensate was sampled directly at the source of the fire, the condensate on the dumps was sampled a few hundred metres from the source, and the snow was sampled a few hundred metres from the source. The lowest concentrations were observed in the snow cover and the highest in the preliminary condensates.
Assuming that the emissions of TPS condense in the same way as in the coal combustion experiment, the comparison of the condensates and the dissolved part of the snow allows us to estimate the mobility of elements in the air/snow system (Ti <Fe <Ga <V <Ni <Sr <Rb <Mn <Ca <Pb <Cd <Cu <K <Zn <Cr <Na <Mg <As). It was also possible to estimate TE migration in the gas-aerosol phase during self-heating and combustion of coal waste. In addition, physico-chemical models of elements mobility and minerals deposition were developed.
1. Introduction
There are many environmental problems associated with coal mining. One of them is the oxidation of the sulphides contained in the coal and the production of acid mine drainage, which flows into a river or lake and kills everything that lives. No less acute problem is the utilisation of coal in man-made mountains of "empty" rock – heaps. These "empty" rocks form heaps up to 60-80 m and dumps spinal form (in the amount of 92%), less – flat dumps (8%). The lithological composition of the dumps reflects the composition of the coal-bearing strata and consists of argillite (60-80%), siltstone (10-30%), sandstone (4-10%), limestone (rarely up to 6%, typically less), as well as significant coal impurities (6-20%). In addition, the quarries occupy large living spaces and fill the air with dust , , . The main problem, as we see it, is the spontaneous combustion of coal seams and waste, which is linked to atmospheric pollution.
1.1. Highlights
During coal combustion, not only As and Sb are volatilized, but also Ti, Fe.
The mobility of elements in the air-snow system is calculated.
The formation of a wide range of minerals in a burning coal waste is shown.
1.2. Coal and coal wastes fires
Coal and coal waste fires are a major problem around the world. Such fires have a wide range of negative environmental impacts. Coal fires around the world are an environmental disaster characterised by the emission of noxious gases, particulate matter and condensation by-products. They emit greenhouse gases – carbon dioxide (CO2) and methane (CH4), carbon monoxide (CO), mercury (Hg) and other toxic substances . Coal flue gas also contains between 40 and 50 different compounds, many of which are toxic and some of which are carcinogenic. Craig Cassells and John van Genderen have suggested that coal fires may account for up to 2-3 per cent of annual atmospheric carbon dioxide emissions, although there are currently no reliable global estimates for the various components of coal combustion gases, particulate matter and mercury. In addition, such fires also contribute ozone-depleting compounds, including ethane, ethane, propane and propene . According to Burtseva et al. 1991 , the fossil fuel component of the anthropogenic input to the atmosphere is quite significant. For example: in percent of the total input to the atmosphere: Co - 98, Cr - 15, Cu - 32, Ni - 77, Sb - 80, Se - 50, V - 85, Zn - 10, Cd - 9, As - 6, Hg - 20.
The nature of the distribution of trace elements (and especially toxic trace elements) between the organic macerals and the mineral admixtures in many respects determines the behaviour of toxic trace elements in coal combustion processes. Thus, organically bound trace elements pass into the gas phase and fly ash, whereas inorganic trace elements pass into bottom ash and slag. An important exception is Hg: regardless of the mode of occurrence of Hg, the majority of Hg in coal passes to the gaseous phase during coal combustion .
Fly ash (FA) is the major combustion residue (typically 60-88%) produced during the combustion of pulverized coal in thermoelectric power plants (TEPs). The chemical classification system of FAs has been organized by Vasilev at all , , , according to the contents, common geochemical associations and significant positive or negative correlations of ash-forming elements in FAs using three end members, namely:
1) sum of Si, Al, K, Ti and P oxides;
2) sum of Ca, Mg, S, Na and Mn oxides;
3) Fe oxide.
This approach identified four chemical FA types (sialic, calsialic, ferricialic, and ferricsialic) with three dominant trends (high acid, medium acid and low acid).
A fascinating list of research on coal and peat fires collected in the books "Coal and Peat Fires: A Global Perspective, Volumes 1-4" edited by G. Stracher, A. Prakash, E. Sokol , , , . It discusses the origin of coal and coal fires; mining and use of coal; combustion and coal petrology; environmental and health impacts of coal fires; combustion by-products; geochemical, geophysical and engineering methods for studying coal fires; control, extinction and policy implications of coal fires; and much more.
1.3. Composition of coal
A great deal of information on the chemical composition of coal has been accumulated over the last few decades. The main chemical constituents of coal are carbon, hydrogen and oxygen, with smaller amounts of nitrogen and sulphur. The chemical formula of coal can be approximated as C135H96O9NS .
In 1990-2000, L.Ya. Kizilshtein worked on organic geochemistry, composition of coal, ecogeochemistry of elements mixtures in coal. He found out that coals contain more than 50 trace elements with different chemical properties. Usually, their amount does not exceed 1%, with the exception of sulphur , , , . He also studied the formation of the gaseous phase of elemental mixtures during coal combustion. Kizilshtein L.Y. with Levchenko S.V. proposed a method of evaluation of ecologically dangerous trace elements in coals burned in thermal power stations. It has been shown that the release of trace elements into the gas phase of the incineration products is an important characteristic for the assessment of atmospheric air pollution .
At the same time, the Australian geochemist D.J. Swaine was studying the trace elements in coal and their distribution during combustion . D.J. Swaine is also best known for his comprehensive studies of the trace element geochemistry of coals. These include the widely cited reference books Trace Elements in Coal and Environmental Aspects of Trace Elements in Coal (with his long-time colleague Fari Goodarzi, , ), and nearly 50 scientific papers dealing mainly with various aspects of coal analysis and trace element determination, the geological and environmental significance of trace elements in coal, and the behavior of trace elements in coal utilisation. One of his suggestions for future work was: speciation in flue gas and stack emissions, especially for arsenic, chromium, mercury and selenium . He also concluded that stack emissions are significant contributors of Mo and Se to the Wallerawang environs, although the amounts are not considered harmful .
In the next decade (2000-2010), Russian geochemists Ya. E. Yudovich and M. P. Ketris collected, analyzed and summarised the trace element data of the world's major coal basins and deposits. As a result of this great work have been calculated average (clark) contents in coals 25 trace elements, which are based on the evaluation of information on concentrations of these elements in coals, and coal affinity indexes (coalphile indexes) , . Particular attention is paid to such elements in coals as mercury, uranium, arsenic, chromium and selenium , , , .
According to Ya. E. Yudovich, the elements can be divided into four groups:
1. Non-coaliferous elements (coal affinity index < 1): I, Cl, Mn, Br, Rb and Cs.
2. Weak or moderately coalfriendly elements (coal affinity indices between 1 and 2): Ti, Zr, F, Cd, V, Ta, Cr, Y, Li and P.
3. Coal-phile elements (coal affinity indices between 2 and 5): Ni, Hf, Sn, La, Co, Ba, Sc, Nb, Sr, Th, Ga, Cu, REE, Zn, W, Au, In, Pb, U, B and Be.
4. Elements with a high affinity for coal (coal affinity index > 5): Ag, Sb, Tl, As, Mo, Ge, Hg, Bi and Se.
The higher the coal affinity index, the greater the contribution of an authigenic fraction of the given trace element (represented by organic or micromineral forms), and the smaller the contribution of a clastogenic fraction (represented by macromineral [e.g. silicatic] forms) .
L.P. Rikhvanov and S.I. Arbuzov have made systematic studies of coal basins and deposits in Russia (Siberia and the Russian Far East) and in neighbouring countries (Kazakhstan and Mongolia) in an attempt to fill this gap and to determine the main regularities of the formation of coals with different radiogeochemical characteristics. They present new data on the geochemistry of U and Th in coals and peats and generalise the extensive knowledge on the radiogeochemistry of coals , , , .
B.V. Chesnokov carried out mineralogical studies of burnt dumps in the Chelyabinsk Coal Basin, and with his team he discovered about 30 new technogenic minerals . Combustion metamorphic events as a result of coal fires in the Chelyabinsk and Kuznezk regions were well studied by Sokol E.V. , , , .
The American A. Kollker is an expert in coal geochemistry and trace element geochemistry, he has studied coal quality, toxic inorganic substances in the environment, and human health effects from energy resources and use. His research focuses on mercury and trace element contents of Ukrainian, South African and USA coals.
1.4. Vapour phase trace element emissions from coal combustion
The problem of coal seam and waste dump fires has been widely studied. However, the question of the gas phase emission of trace elements from coal fires and coal combustion is still open.
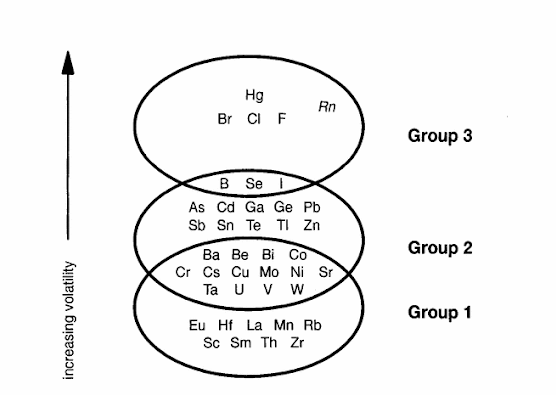
Figure 1 - Categorisation of trace elements based on volatility behavior
Note: by L.B. Clarke [44]
Detailed investigations of the inlet and outlet flows of wet lime FGDs have been reported by H. Gutberlet from Germany and R. Meij from the Netherlands , , . Gaseous compounds condense on particulate matter in the wet FGD unit. In both cases, trace elements were introduced into the FGD system partly through the flue gases, but mainly in the limestone used for desulphurisation. The lime is a significant source of certain trace elements, particularly As, Cd, Pb and Zn, with the lime contributing over 90% of the trace element input . However, flue gases were found to be the main source of volatile trace elements. In the Dutch study , evaporated elements were detected in the gas phase downstream of the ESP before the FGD; В as B203, Hg as Hg° and Se02. A small amount of As was also detected in the gas phase (<1%).
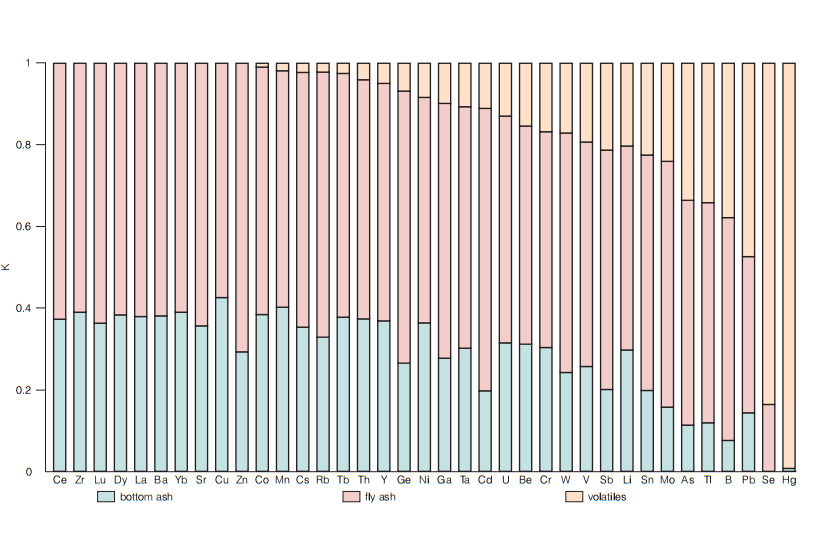
Figure 2 - Trace element partition coefficients in the combustion by-products of a 1050 MW coal-fired power plant
Note: 51
1) trace elements retained in the solid waste;
2) trace elements partially retained in the solid waste;
3) trace elements primarily emitted to the atmosphere.
This classification is similar to those generally used based on enrichment factors and relative enrichment factors , , . This mathematical estimation shows a theoretical possibility of presence of the list of chemical elements (Co, Mn, Cs, Rb, Tb, Th, Y, Ge, Ni, Ga, Ta, Cd, U, Be, Cr, W, V, Sb, Li, Sn, Mo, As, Tl, Pb, Se) in the gas-aerosol phase.
Coal waste fires differ from control fires at TCP. There are no afterburning, scrubbing and refining systems, and the "empty" rocks that form the heaps have an effect on the composition of the burning product. The aim of our investigation is to estimate the TE migration in the gas-aerosol phase during self-heating and combustion of waste heaps competing with TCP emission and experimental coal combustion.
2. Materials and methods
2.1. Study object
The objects of the study were steam-gas condensate from experimental coal burning, steam-gas condensate selected on burning coal dumps, snow cover in the zones of influence of TPS-2, TPS-3 in Novosibirsk (Fig. 3.).
Initially, we studied burning coal dumps, but it was impossible to estimate the degree of pollution from the data obtained. Therefore, we decided to conduct a coal burning experiment and use our data on snow cover composition in the vicinity of the TPS.
To study high-temperature technogenic processes, we decided to use two main sampling methods: 1) condensation of the gas-aerosol phase directly at the emission source (0-1 m); 2) selection of snow samples at a distance from the source (0.3-2 km). The selection of vapour-gas condensates is the most representative sampling method for the study of high temperature gases. This method has been used for several decades to obtain condensates of natural volcanic gases . The study of snow cover has also been used for several decades to obtain data on the arrival of pollutants from the atmosphere to the underlying surface, since snow, as a natural plate accumulator, gives the actual value of dry and wet fallout in the cold season .
The coal waste dumps studied are located in the Chelyabinsk and Kemerovo regions (Fig. 3 a, b). Waste from the Podozernaya mine near the town of Kopeisk (Chelyabinsk region) was stored (1953-1970) in heaps about 60 m high, with a surface area of 68160 m2 and a volume of 1200000 m3. This waste has been incinerated since 1984. The waste heaps of the Chelyabinsk Oblast consist of up to 80% mudstone, 5-7% siderite, 1-2% siltstone, 5-15% coal, and often contain sulphides of iron and other elements .
The Bochatsky open-cast mine is located in the Kemerovo region of Russia. Spontaneous combustion is also observed in the waste and working face of the open pit. The basis of the substrate of the Bochatsky opencast mine dumps are overburden and bedrock. The most common are sandstones and siltstones (70%). In addition, the tailings contain up to 10% coal. The predominant cement is argillaceous, its quantity varies from 5-7 to 10-18%. Carbonate cement usually has calcite and dolomite composition, its amount varies from 20 to 30%. The Bochatsky opencast mine has 5 refuse tips, some of which are no longer in use (North and West), and others (East, Sagarlik and Buldozerny) are still in operation. Coal fires are observed in all of them.
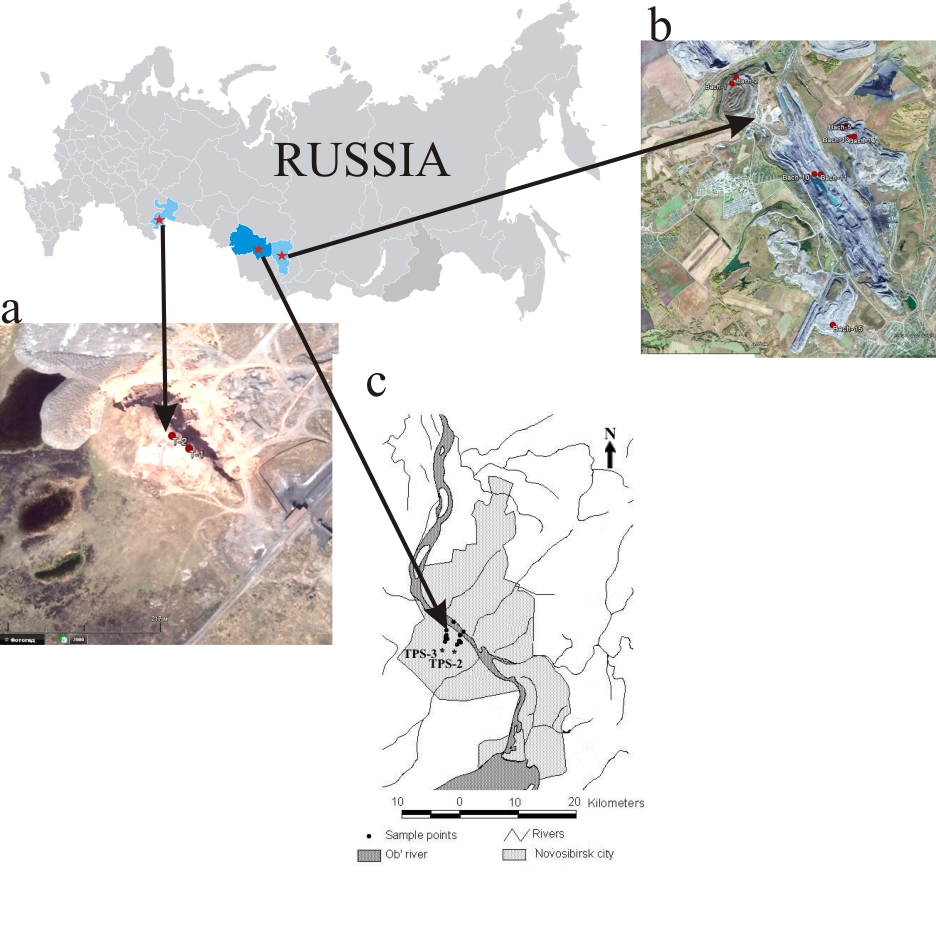
Figure 3 - Geographical location of the research object:
a - Chelyabinsk region; b - Kemerovo region; c - Altai Territory
Methods of investigation include the following:
- Using the collection of steam condensates on waste heaps and solid sampling for chemical analyses and experiments.
- Using the collection of snow cover near TPS -2 and TPS-3 in Novosibirsk city.
- Thermodynamic modelling
2.2.1 Field investigations
A set of gas condensate samples was collected from the burning slag heaps in the Chelyabinsk region (Podozernaya mine), coal dumps in the Kemerovo region (Bachatskij open-cast mine). The location of the sampling points is shown in Figure 3. Gas is emitted slowly through small holes (5 to 10 cm in diameter) at the top of the heaps. We used a standard condensate sampling method that is widely used for volcanic gas studies. Gas was passed through a jet-heated Teflon funnel, then through a flexible Teflon tube connected to a bubbler trap cooled by cold water (Fig 4a). A small hand pump was used to introduce the gas into the trap , . Waste rock samples were also collected.
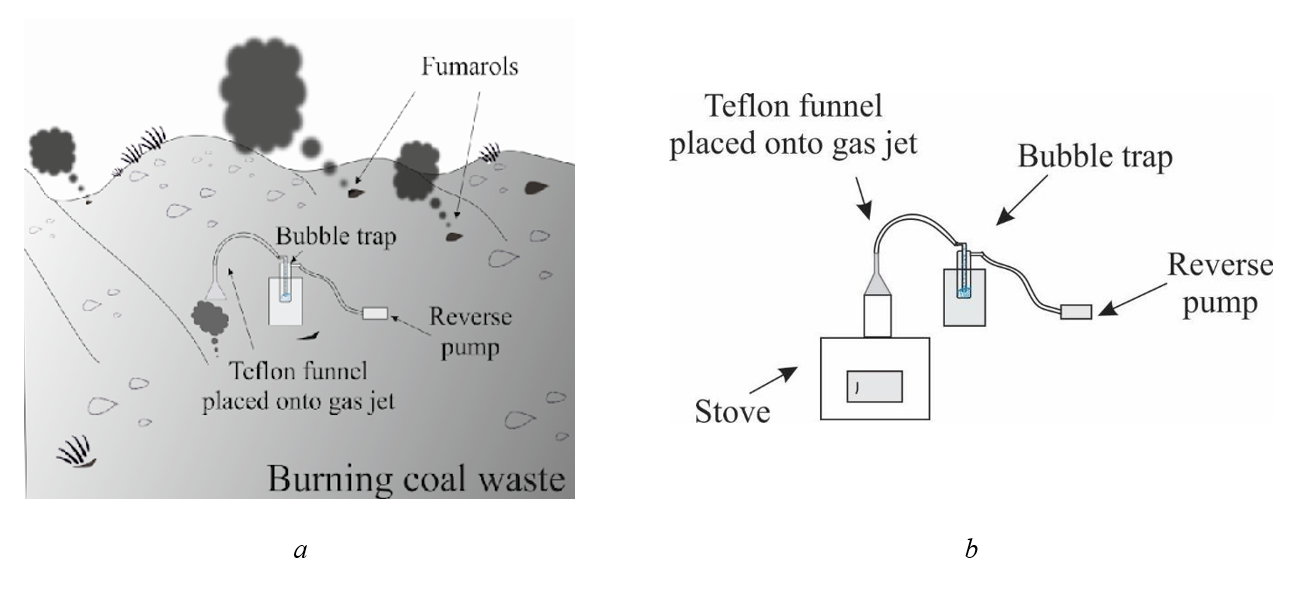
Figure 4 - Schematic of the experiments on condensate collection:
a – on the burning coal waste; b – in the laboratory
"Old fires" were observed in the upper part of the Podozernaya mine waste heap, in the lower part of the Bachatsky open-cast mine and in the upper part of the northern waste hip (Bachatsky open-cast mine).
Three types of coal from the Kuznetsk coalfield were used for the coal combustion experiment. The experiment was carried out according to the following scheme. Coal was burned in a furnace and the gases were discharged through the attached flexible Teflon tube into a bubbler trap cooled by liquid nitrogen (Fig. 4b).
Snow cover was sampled in the zones of influence of the TPSs (Fig. 6). The investigated TPSs are located within the urban development in the north-western part of the city of Novosibirsk. The fuel for the TPSs is Kuznetsk coal. Sampling points were located in open areas of the area. Samples were selected taking into account the mid-winter wind rose for the surface atmosphere layer. In this direction, the maximum of TPS emissions was recorded . A standard procedure was used to select snow .
2.2.3. Chemical analysis
The multielement composition of the condensates and snowmelt water was analyzed by the ICP-AES method, IRIS Advantage spectrometer (Thermo Jarell Ash Corporation, United States) at the Analytical Centre of the Institute of Geology and Mineralogy, Sb RAS. The determination error is 5-10%.
Concentrations of major, minor, and trace elements in the water samples were determined by inductively coupled plasma mass spectrometry (ELAN-9000 DRC-e, Perkin Elmer, Shelton, USA). Accuracy and precision were estimated to be 7% or better at the mg/L concentration level and 10% or better at the µg/L concentration level. Reference standard material (RSM) used in the QC of the ICP-MS analytical results for liquids is certified wastewater – trace metals solution (CWW-TM-D, High Purity Standards, Charleston, USA), and for solids – simulated matrix reference material (CRM-S-I, High Purity Standards, Charleston, USA).
2.2.4. Thermodynamic modeling methods
A thermodynamic model of the anthropogenic body formation in the combustion conditions was developed. The calculations were carried out with the help of the "Selector" PC (57). The technique is based on the principle of a flow-through step reactor and covers the area of formation of technogenic minerals, gases and aerosols during the interaction of heated rocks with atmospheric air and water at a constant pressure of 1 atm. А portion of the fluid (mobile components, in our case gas, solution) enters the reactor from the environment; the fluid interacts with the rock, changes the rock and changes itself, the thermodynamic equilibrium. The newly formed fluid enters the next reactor, where it reacts again with the rock, etc. The time simulation is created by several portions of the passage of the primary fluid from the environment, which reacts with the already altered previous portions of the rock. In the model, this is defined as "time". Calculations were carried out for the multisystem H-O-N-S-Si-Ti-Al-C-Cl-FK-Mg-Na-Fe-Cu-Zn-Ni-Pb-Rb-Sr-Ba-Li-V -Mn-Be-Cr-As-Co-Cs in the temperature range 50-450 ° C (T1-high-temperature model, that simulated "old fires"), 50-200 ° C (T2-low-temperature model, that simulated "young fires"). Each of the models evolves in time; calculations were made for ten "times". To calculate the thermodynamic properties of substances, standard databases of components of aqueous solution, gases, melts, solids and minerals were used .
3. Results
3.1.Burning coal dumps
Steam and gas condensates, collected on burning coal dumps, had a largely different chemical composition. This is due to the age of the fires. When the coal waste dumps burn for decades, the metamorphic processes inside them lead to the formation of new mineral phases and the emission of a specific range of elements. The metamorphic processes in burning dumps have been studied in detail by Chesnokov B.V. and Sokol E.V. , , .
According to mean element concentrations in the gas condensates sampled in situ, the basic elements of the slag is Sulfur (more than 100 ppm). K > Ca > Na (100-10 ppm). Al > Fe > Mg (10-1 ppm). Zn > Ti > As > Ba > Pb > Sn > Mn > Cu (1-0.1ppm) Cr > Cd > Sr > Co (0.1 – 0.01 ppm). As (less than 0.001 ppm) (Table 1). In a Table 1 probes with names Bach 1 – Bach 15 are from Bachatsky open-cut mine. And probes with the names P1-P5 are from waste hip of Podozernaya mine. In a Table 1 also shows the age of the fires from which the samples were taken. 6 samples were taken from “old fires”, 5 samples from “young” ones.
Since the initial material of the dumps differs in chemical composition and duration of combustion, we observe different compositions of gases. In order to compare the compositions of gases from the burning dumps of the Podzernaya mine, we took the gas compositions only from the "old fires" at the Bachatsky coal mine (fig. 5). Because it is more correct. Steam gas condensates obtained from the dumps of Podozernaya mine have higher concentrations of almost all of the measured chemical elements. Exceptions are: P, Sr, Ba. Concentrations of the same order are observed for S, Na, Mn, Sn, Zn.
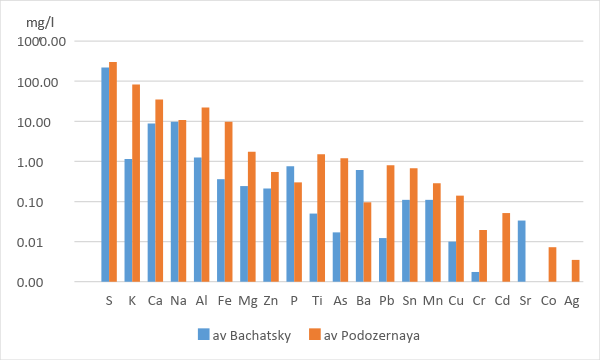
Figure 5 - Average element concentrations in the condensates of "old fires" at the dumps of the Bachatsky coal mine and at the dump of the Podozernaya coal mine
The detection of measurable amounts of elements in the air raises questions about their sources, mechanisms of migration in the emission streams and comparative mobility. It is impossible to take into account the many factors that determine the emission of these chemical elements from the tailings in these field conditions. A series of laboratory experiments have been carried out to rule out errors in condensate collection and the cumulative effect of air pumping.
Table 1 - Concentration of elementsgas condensates, collected on burning coal dumps
Probe | Bach 1, ppm | Bach 2, ppm | Bach 5, ppm | Bach 6, ppm | Bach10, ppm | Bach 11, ppm | Bach 13, ppm | Bach 14, ppm | Bach 15, ppm | P 1, ppm | P 5, ppm |
"Old fires" | "Old fires" | "Young fires" | "Young fires" | "Old fires" | "Old fires" | "Young fires" | "Young fires" | "Young fires" | "Old fires" | "Old fires" | |
S | 510 | 185 | <0.1 | <0.1 | 122 | 60 | 7 | 0.1 | 1.1 | 200 | 400 |
K | 2.2 | 1.4 | 1.7 | 9.1 | 0.5 | 0.5 | 0.8 | 4.4 | 3.9 | 130 | 35 |
Ca | 12 | 5 | 12 | 1.7 | 10 | 8 | 2 | 1 | 6 | 45 | 25 |
Na | 10 | 9 | 1 | 1.5 | 9 | 11 | 1 | 1 | 10 | 1.5 | 20 |
Al | 1.2 | 0.7 | 0.11 | 0.13 | 2.1 | 1 | 0.1 | 0.1 | 0.8 | 2 | 42 |
Fe | 0.6 | <0.1 | 0.04 | 0.011 | 0.25 | 0.23 | 0.24 | 0.01 | 0.1 | 3.5 | 16 |
Mg | 0.31 | 0.16 | 0.19 | 0.07 | 0.27 | 0.23 | 0.08 | 0.05 | 0.11 | 3 | 0.50 |
Zn | 0.15 | <0.1 | 0.17 | 0.01 | 0.42 | 0.06 | 0.04 | <0.1 | <0.1 | 0.29 | 0.80 |
P | 1.2 | 0.9 | 0.04 | 0.1 | 0.6 | 0.32 | 0.03 | 0.06 | 0.42 | 0.13 | 0.47 |
Ti | <0.01 | 0.05 | <0.01 | <0.01 | <0.01 | <0.01 | <0.01 | <0.01 | <0.01 | <0.01 | 1.5 |
As | <0.01 | 0.01 | <0.01 | <0.01 | <0.01 | 0.024 | <0.01 | <0.01 | 0.024 | <0.01 | 1.2 |
Ba | 0.76 | 0.41 | 0.11 | 0.10 | 0.69 | 0.58 | 0.096 | 0.05 | 0.68 | 0.13 | 0.061 |
Pb | 0.03 | 0.0087 | 0.020 | 0.0005 | 0.0023 | 0.008 | 0.0012 | 0.0009 | 0.0063 | 0.0055 | 1.6 |
Sn | <0.001 | <0.001 | 0.036 | 0.01 | 0.11 | <0.001 | 0.021 | <0.001 | 0.048 | <0.001 | 0.67 |
Mn | 0.11 | <0.03 | 0.01 | <0.03 | <0.03 | <0.03 | <0.03 | <0.03 | <0.03 | 0.27 | 0.30 |
Cu | 0.01 | <0.002 | 0.0033 | <0.002 | <0.002 | <0.002 | <0.002 | <0.002 | <0.002 | <0.002 | 0.14 |
Cr | 0.001 | 0.002 | 0.010 | 0.001 | 0.002 | 0.002 | 0.002 | 0.006 | 0.002 | 0.007 | 0.032 |
Cd | <0.01 | <0.01 | <0.01 | <0.01 | <0.01 | <0.01 | <0.01 | 0.00048 | <0.01 | 0.008 | 0.095 |
Sr | 0.033 | 0.025 | 0.049 | 0.0066 | 0.036 | 0.04 | 0.0101 | 0.0039 | 0.027 | <0.01 | <0.01 |
Co | <0.0001 | <0.0001 | 0.0003 | <0.0001 | <0.0001 | <0.0001 | <0.0001 | 0.0002 | <0.0001 | 0.0060 | 0.0085 |
Ag | <0.001 | <0.001 | 0.0009 | 0.0053 | <0.001 | <0.001 | 0.0005 | 0.0014 | 0.0023 | 0.0038 | 0.0032 |
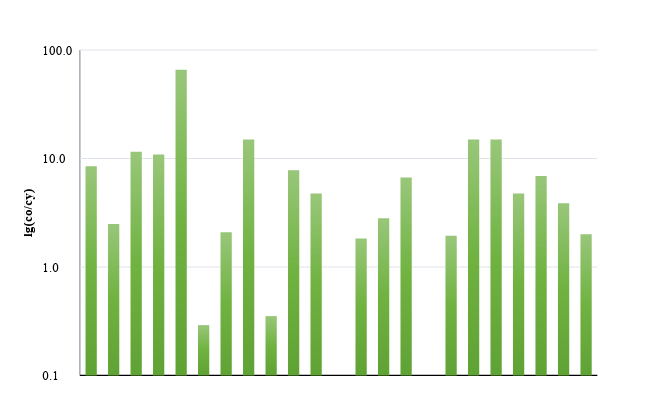
Figure 6 - The ratio of elements concentrations in condensates of the "old" and "young fires" at the coal dumps
For the experiment we took 3 types of coal from Kuzbas coal basin (It is Bachatsky coal mine includes). These 3 types of coal differ a little bit in their physical properties: colour, gloss, granularity. Vapour condensates obtained by combustion of these coals have practically identical composition.
It contained macro-components (Ca, Na, Mg, K in the concentration of 5-60 ppm), trace elements Zn, Al, As, Sr, Fe (0.1-0.01 ppm), Ba, Mn, Cu, Ni, Ti, Pb (0.01-0.001 ppm) and Cr, Rb, V, Sb, Cd, Co, Sn, Ga (less than 0.001 ppm). (Table 2)
Concentrations of elements in gas condensates obtained by experiment are the less exceed the concentrations of elements collected on burning coal dumps. It is worth noting the high content of the main rock-forming elements Ca, Na, Mg, K in condensates obtained by experiment. This is discussed in more detail in Chapter 4 (Discussion).
It is very difficult to estimate the degree of air pollution based on the composition of steam-gas condensates. Therefore, we decided to make a comparison with the composition of the snow cover in the vicinity of the Novosibirsk TPS. This used the Kusnezk coals, as in our coal combustion experiment.
Table 2 - Concentrations of elements in gas condensates, collected by tentative combustion
N | Coal 1, ppm | Coal 2, ppm | Coal 3, ppm |
Ca | 53 | 61 | 67 |
Na | 11 | 11 | 17 |
Mg | 8.1 | 11 | 12 |
K | 4.8 | 5.4 | 15 |
Zn | 0.58 | 0.63 | 0.17 |
As | 0.13 | 0.096 | 0.4 |
Fe | 0.18 | 0.23 | 0.11 |
Sr | 0.12 | 0.17 | 0.23 |
Mn | 0.07 | 0.09 | 0.07 |
Cu | 0.01 | 0.16 | 0.06 |
Pb | 0.019 | 0.012 | 0.009 |
Ti | - | 0.01 | - |
Ni | 0.002 | 0.006 | 0.011 |
Cr | 0.005 | 0.007 | 0.003 |
Rb | 0.003 | 0.004 | 0.006 |
V | 0.001 | 0.005 | - |
Cd | 0.002 | 0.002 | 0.003 |
Sb | 0.001 | 0.001 | 0.004 |
Ga | 0.0004 | 0.0006 | - |
3.3. TPS gas emissions
In snow samples, the dissolved fraction (obtained by careful filtration of melting snow) was analyzed. In general, the composition of the dissolved part of the snow samples is similar to that of natural surface water. In the dissolved part of the snow, emissions from CHP plants are transported in the form of aerosols and vapour-gas phase. The mechanism of behaviour of pollutants in snow during melting is not sufficiently studied, lower concentrations of technogenic and rock-forming elements in the dissolved part of the snow sample are explained by the processes of sorption of these elements into larger particles of the aerosol and finding them predominantly in the suspended part.
It contained macro components (Ca, K, Na, Mg with the concentration of 0.1-15 ppm), trace elements S, Zn, Ti, B (0.1-0. 01 ppm), Mn, Br, Cu, Sb, As, Ni, Zr, V, Pb (0.01-0.001 ppm) and I, U, Rb, Sn, Mo, Se, Ga Cr, Cd, Th, Ge (less than 0.001 ppm) (Table 3). Of these, Sb, U and Ge are the most prominent; they exceed background levels by a factor of 5-10 and are toxic and radioactive (U) elements. Sn, Nb and Mo are also present in high concentrations.
The concentrations of elements exceeding the MPC for water in Table 10 are shown in bold. A number of samples exceed the MPC for K, Ca, Na, Fe, Mn, Cu.
Table 3 - Content of elements in the dissolved part of the snow cover in the area of TPS-2 and TPS-3
N | 1 | 2 | 3 | 4 | 5 | 6 | 7 | 8 | 9 | 10 | 11 | 12 | 13 | 14 | 15 | 16 |
Na, ppm | 0.6 | 0.26 | 1.64 | - | 0.42 | 0.23 | 0.31 | 0.37 | 0.3 | 0.26 | 0.19 | 0.17 | 0.27 | 0.59 | 0.3 | 0.17 |
K, ppm | 0.42 | 0.24 | 0.71 | 2.21 | 0.36 | 0.67 | 0.42 | 0.56 | 0.99 | 0.35 | 0.39 | 0.34 | 0.52 | 0.21 | 0.37 | 0.2 |
Ca, ppm | 5.9 | 3.7 | 3.7 | 14.6 | 4.8 | 5 | 9.2 | 15 | 10.7 | 2.9 | 1.8 | 4.4 | 3.8 | 2.9 | 3.2 | 1.5 |
Ti, ppm | 0.009 | 0.021 | - | - | 0.006 | 0.02 | 0.005 | - | 0.058 | 0 | 0.008 | 0.01 | 0.009 | 0.005 | 0.004 | 0.006 |
Fe, ppm | 0.06 | 0.23 | 0.02 | 0.11 | 0.02 | 0.19 | 0.072 | 0.11 | 0.6 | 0.054 | 0.09 | 0.23 | 0.11 | 0.08 | 0.062 | 0.044 |
Mn, ppm | 0.006 | 0.01 | 0.003 | 0.005 | 0.02 | 0.01 | 0.005 | 0.005 | 0.024 | 0.006 | 0.003 | 0.01 | 0.01 | 0.005 | 0.005 | 0.006 |
Mg, ppm | 0.13 | 0.13 | 0.4 | - | 0.13 | 0.12 | 0.2 | 0.4 | 0.19 | 0.17 | 0.2 | 0.1 | 0.16 | 0.41 | 0.19 | 0.12 |
Ga, ppm | 0.00024 | 0.00022 | 0.00012 | 0.0004 | 0.0001 | 0.0004 | 0.0002 | 0.0004 | 0.0007 | 0.0002 | 0.0001 | 0.0003 | 0.0002 | 0.0002 | 0.0003 | 0.00006 |
Ge, ppm | 0.00014 | 0.00005 | 0.00008 | 0.00026 | 0.0001 | 0.0001 | 0.0001 | 0.0001 | 0.0003 | 0.0001 | 0.0001 | 0.0002 | 0.0001 | 0.0001 | 0.0001 | 0.00002 |
Se, ppm | 0.00015 | 0.00021 | 0.0002 | 0.00028 | 0.0002 | 0.0005 | 0.0002 | 0.0004 | 0.0003 | 0.0003 | 0.0001 | 0.0002 | 0.0003 | 0.0002 | 0.0002 | 0.00006 |
Rb, ppm | 0.0005 | 0.0008 | 0.0006 | 0.0018 | 0.0006 | 0.0013 | 0.0008 | 0.0007 | 0.001 | 0.0007 | 0.0004 | 0.0003 | 0.0007 | 0.0004 | 0.0009 | 0.0002 |
Sr, ppm | 0.02 | 0.03 | 0.03 | 0.05 | 0.04 | 0.03 | 0.03 | 0.09 | 0.06 | 0.04 | 0.03 | 0.02 | 0.03 | 0.04 | 0.04 | 0.009 |
Y, ppm | 0.00006 | 0.00016 | 0.00004 | 0.00006 | 0.00005 | 0.00024 | 0.00012 | 0.00016 | 0.00026 | 0.00014 | 0.00011 | 0.00006 | 0.00011 | 0.00008 | 0.00016 | 0.00002 |
Zr, ppm | 0.0004 | 0.0018 | 0.0004 | 0.0003 | 0.0006 | 0.0042 | 0.0015 | 0.0017 | 0.0021 | 0.0012 | 0.001 | 0.0008 | 0.0038 | 0.0015 | 0.0017 | 0.0001 |
Nb, ppm | 0.00001 | 0.00059 | 0.00002 | 0.00002 | - | 0.0001 | 0.00005 | - | 0.0001 | 0.00002 | 0.00004 | 0.00003 | 0.00003 | 0.00001 | 0.00003 | 0 |
Th, ppm | 0.0001 | 0.0005 | 0.0001 | 0.0001 | 0.0002 | 0.0001 | 0.0001 | 0.0003 | 0.0002 | 0.0001 | 0.0001 | 0 | 0.0001 | 0.0001 | 0.0002 | 0.00001 |
U, ppm | 0 | 0.006 | 0.0001 | 0.0001 | - | 0.00004 | - | - | - | - | - | 0.00003 | - | 0.00003 | - | - |
Si, ppm | 0.04 | 0.06 | 0.19 | - | 0.05 | 0.11 | 0.43 | 0.15 | 0.07 | 0.08 | 0.07 | 0.02 | 0.06 | 0.11 | 0.65 | 0.03 |
Pb, ppm | 0.001 | 0.002 | 0 | 0.001 | 0.001 | 0.002 | 0.001 | 0.001 | 0.003 | 0.002 | 0.001 | 0.001 | 0.001 | 0.001 | 0.001 | 0.001 |
V, ppm | 0.0007 | 0.0023 | 0.0007 | 0.0023 | 0.0013 | 0.0011 | 0.001 | 0.0028 | 0.0017 | - | 0.0008 | 0.0013 | 0.0011 | 0.0011 | 0.0016 | 0.0004 |
Cr, ppm | 0.00022 | 0.00008 | - | - | - | - | 0.00009 |
| 0.00059 | - | - | 0.00046 | - | 0.00006 | 0.00005 | 0.00007 |
Ni, ppm | 0.002 | 0.0023 | 0.0019 | 0.005 | 0.0035 | 0.0038 | 0.0011 | 0.0021 | 0.006 | 0.0009 | 0.001 | 0.0017 | 0.0019 | 0.0009 | 0.0015 | 0.0021 |
Cu, ppm | 0.003 | 0.003 | 0.003 | 0.007 | 0.002 | 0.004 | 0.003 | 0.002 | 0.007 | 0.002 | 0.001 | 0.037 | 0.002 | 0.008 | 0.004 | 0.001 |
Zn, ppm | 0.016 | 0.026 | 0.003 | 0.07 | 0.009 | 0.014 | 0.014 | 0.009 | 0.032 | 0.007 | 0.006 | 0.056 | 0.017 | 0.008 | 0.031 | 0.009 |
Ag, ppm | 0.00004 | 0.00007 | 0.00003 | 0.00001 | 0.00001 | 0.00003 | 0.00001 | 0.00006 | 0.00023 | 0.00001 | 0.00001 | 0.00003 | 0.00002 | 0.00002 | 0.00003 | 0.00001 |
Cd, ppm | 0.00021 | 0.00043 | 0.00009 | 0.00028 | 0.00021 | 0.00024 | 0.00032 | 0.00018 | 0.00026 | 0.00009 | 0.00004 | 0.00008 | 0.00012 | 0.00009 | 0.00011 | 0.00002 |
Br, ppm | 0.006 | 0.005 | 0.007 | 0.018 | 0.008 | 0.008 | 0.005 | 0.006 | 0.011 | 0.007 | 0.004 | 0.004 | 0.007 | 0.007 | 0.008 | 0.003 |
Mo, ppm | 0.0002 | 0.0005 | 0.0004 | 0.0008 | 0.0004 | 0.0003 | 0.0005 | 0.0003 | 0.0004 | 0.0004 | 0.0002 | 0.0002 | 0.0003 | 0.0004 | 0.0003 | 0.0001 |
Sn, ppm | 0.0002 | 0.0012 | 0.0001 | 0.00003 | 0.00004 | 0.0008 | 0.0004 | 0.0003 | 0.0012 | 0.0007 | 0.0003 | 0.0002 | 0.0003 | 0.001 | 0.0001 | 0.0001 |
Sb, ppm | 0.001 | 0.002 | 0.002 | 0.002 | 0.001 | 0.002 | 0.002 | 0.002 | 0.005 | 0.006 | 0.002 | 0.002 | 0.008 | 0.005 | 0.011 | 0.001 |
S, ppm | 0.07 | 0.06 | 0.07 | 0.38 | 0.07 | 0.1 | 0.06 | 0.14 | 0.17 | 0.07 | 0.02 | 0.09 | 0.07 | 0.08 | 0.04 | 0.03 |
I, ppm | 0.0007 | 0.0006 | 0.0012 | 0.0032 | 0.0021 | 0.0008 | 0.0009 | 0.0005 | 0.0008 | 0.0009 | 0.0004 | 0.0005 | 0.0006 | 0.0006 | 0.0011 | 0.0002 |
As, ppm | 0.0036 | 0.0038 | 0.0025 | 0.0047 | 0.0034 | 0.0044 | 0.0026 | 0.0021 | 0.0039 | 0.0027 | 0.0009 | 0.0018 | 0.0025 | 0.0019 | 0.0068 | 0.0009 |
B, ppm | 0.007 | 0.007 | 0.022 | - | 0.007 | 0.006 | 0.018 | 0.016 | 0.008 | 0.008 | 0.004 | 0.004 | 0.006 | 0.024 | 0.008 | 0.009 |
Note: bold marks the excess of MPC for water
4. Discussion
Assuming that the emissions of TPS occur in the same way as in the coal combustion experiment, the comparison of the condensates and the dissolved part of the snow allows us to estimate the mobility of the elements in the air/snow system.
A direct comparison of these compounds has shown that the main rock-forming elements (Ti, Fe, Ga, V, Ni, Sr, Rb) in the condensate and the snow cover have concentrations of the same order of magnitude (Table 4) and mobility coefficients < 1. The other elements (Ca, Na, Mg, Mn, K, Cu, Zn, As) have concentrations 1 or 2 orders of magnitude higher in the condensate than in the dissolved part of the snow cover (Kmobility > 1). This suggests that this group of elements was also transported in the gas phase, but interacted and redistributed in the system dissolved/weighted snowpack due to a higher migratory ability.
Comparison of the chemical composition of all three objects has shown that the character of the element distribution in the dissolved part of the snow cover near TPS, in condensates sampled on burning coal dumps and in condensates obtained by the experiment is the same (Fig. 7). In the tentative experiment, the condensate was sampled directly at the source of the fire, the condensate on the dumps was sampled a few tens of metres from the source, and the snow was sampled a few hundred metres from the source. The lowest concentrations were found in the snow cover and the highest in the pile condensates. Thus, the chemical composition of the snow cover in the vicinity of TPSs is primarily determined by the composition of the coal used. Regional and global aerosol pollutants have a minimal effect.
Table 4 - Average element concentrations in the dissolved part of the snow cover and in the condensate sampled by the experiment and the mobility coefficients
| Snow. dissolved part, mg/L | Condensate experiment, mg/L | К mobility K=log(Ccond/Csnow) |
Ti | 0.01 | 0.01 | 0 |
Fe | 0.13 | 0.2 | 0.2 |
Ga | 0.0003 | 0.0004 | 0.1 |
V | 0.001 | 0.003 | 0.5 |
Ni | 0.002 | 0.006 | 0.5 |
Sr | 0.037 | 0.17 | 0.7 |
Rb | 0.001 | 0.004 | 0.6 |
Mn | 0.008 | 0.077 | 1.0 |
Ca | 5.8 | 60 | 1.0 |
Pb | 0.001 | 0.013 | 1.1 |
Cd | 0.0002 | 0.002 | 1.0 |
Cu | 0.006 | 0.077 | 1.1 |
K | 0.56 | 8.4 | 1.2 |
Zn | 0.02 | 0.46 | 1.4 |
Cr | 0.0002 | 0.005 | 1.4 |
Nа | 0.4 | 13 | 1.5 |
Mg | 0.2 | 10 | 1.7 |
As | 0.003 | 0.2 | 1.8 |
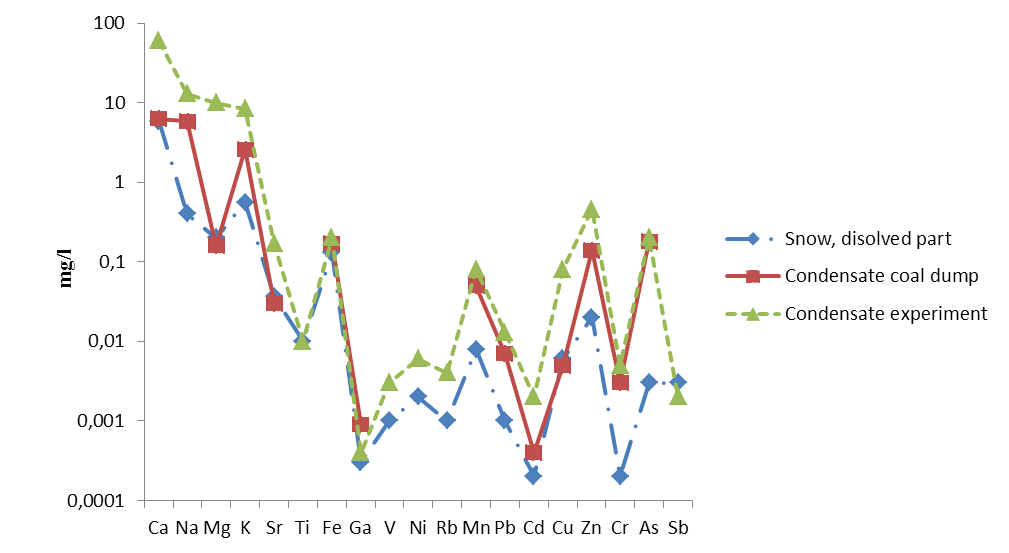
Figure 7 - Comparison of condensate composition obtained from the experimental coal combustion, condensates sampled on burning coal dumps and the dissolved part of the snow cover near coal TPSs
A thermodynamic model of anthropogenic body formation under combustion conditions has been developed. It is based on the principle of a flow-through step reactor and covers the formation of technogenic minerals, gases and aerosols during the interaction of heated rocks with atmospheric air and water at a constant pressure of 1 atm. The natural prototype of the modelled objects was considered to be the technogenic system developing in a burning coal dump in the Chelyabinsk Oblast. The contents of chemical elements (in the waste rocks of the coal dump and in the steam-gas condensates) were taken as input data for modeling. Two variants of the model were calculated at different temperatures. The calculations were carried out with the help of the "Selector" PC (57) for the multisystem H-O-N-S-Si-Ti-Al-C-Cl-FK-Mg-Na-Fe-Cu-Zn-Ni-Pb-Rb-Sr-Ba-Li-V-Mn-Be-Cr-As-Co-Cs in the temperature range 50-450 °C (T1-high temperature model), 50-200 °C (T2-low temperature model). Each of the models evolves in time, calculations were made for ten "times". Standard databases of components of aqueous solutions, gases, melts, solids and minerals were used to calculate the thermodynamic properties of the substances (58-65).
For the simulation, we used the scheme shown in Fig.8
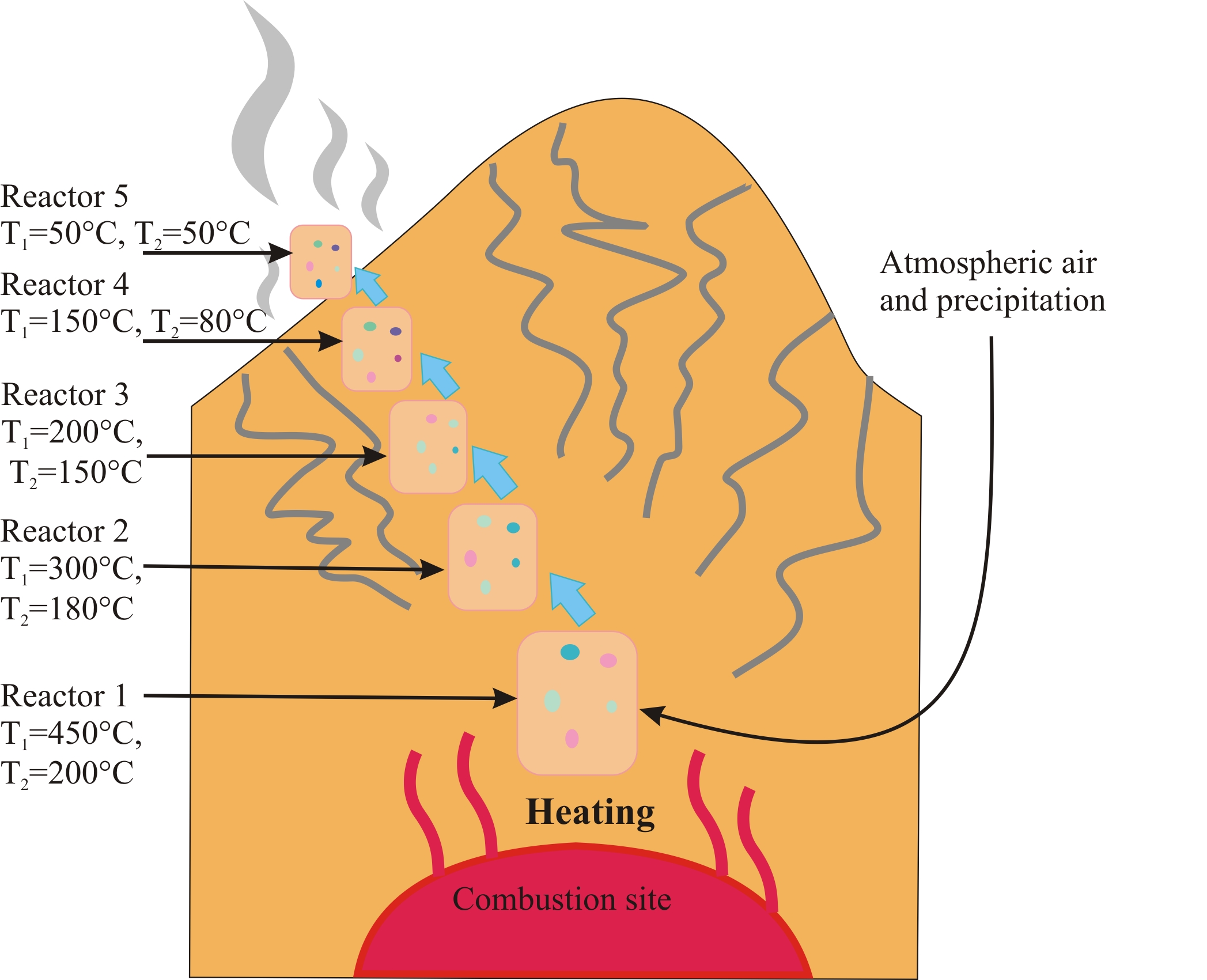
Figure 8 - Scheme of the technogenic system for thermodynamic model
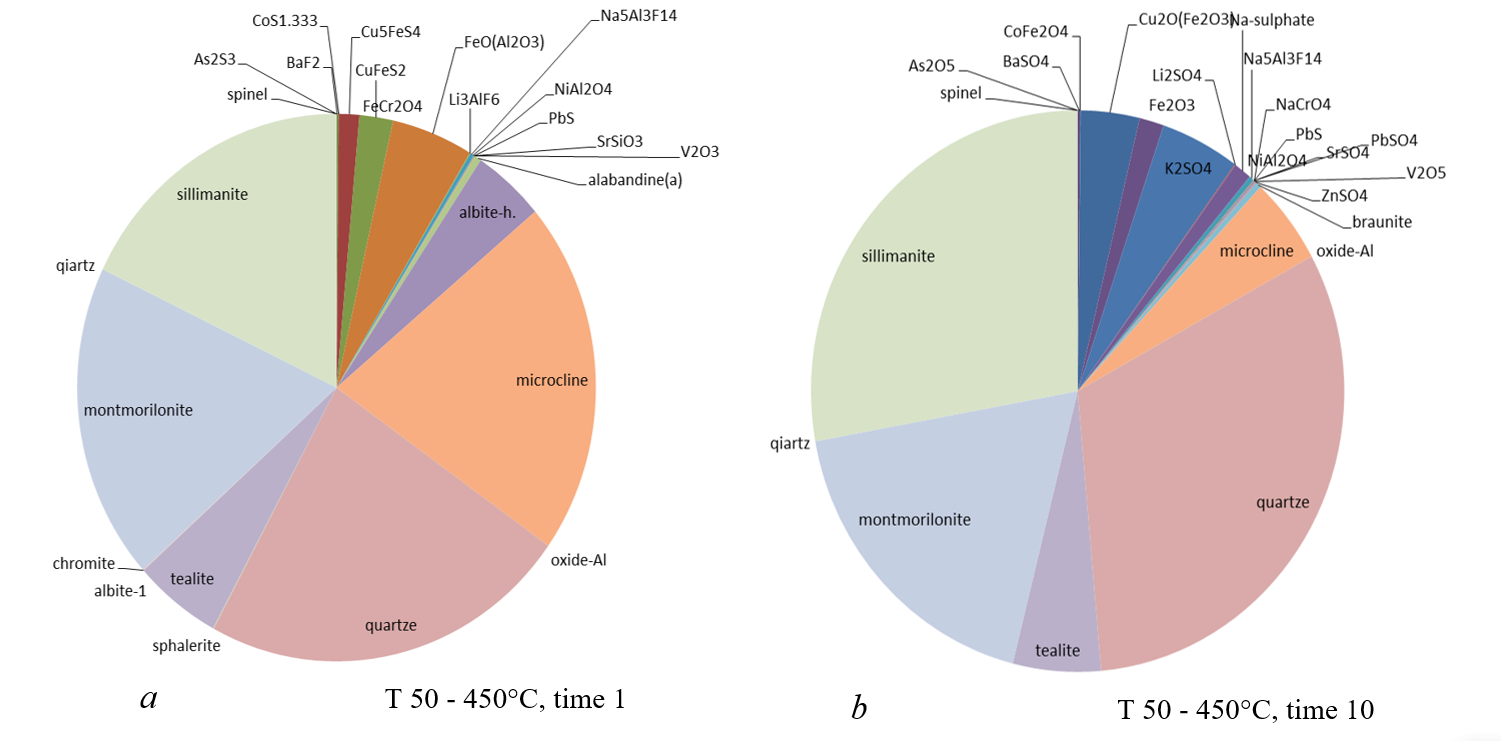
Figure 9 - Mineral composition of the high-temperature system at different times
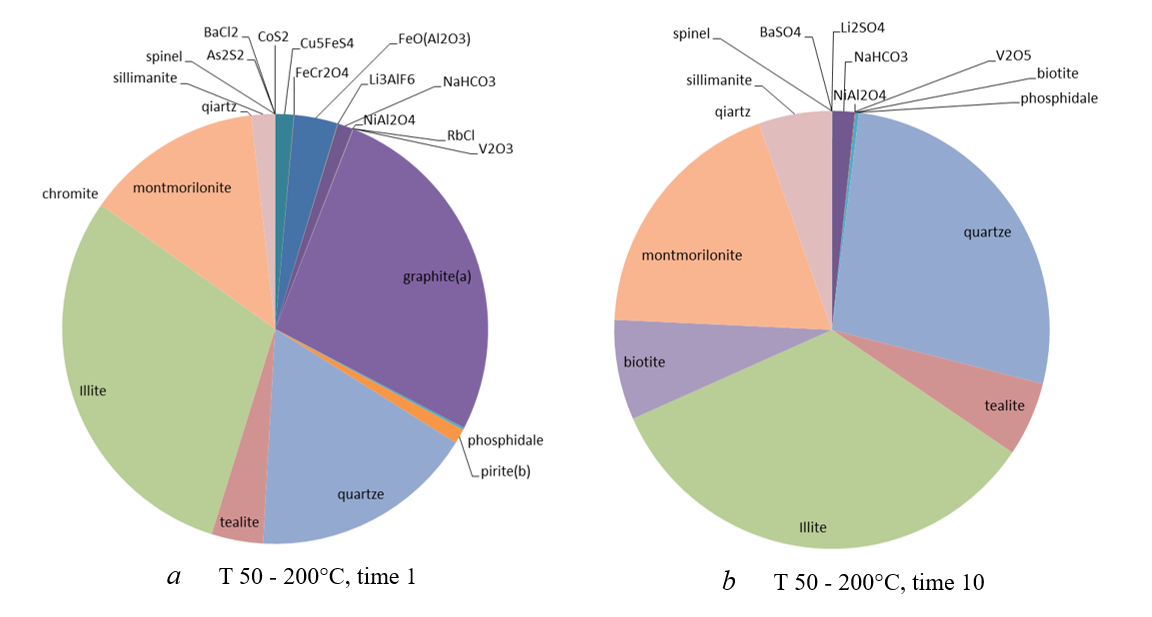
Figure 10 - Mineral composition of the low-temperature system at different times
Thus, depending on the initial temperature of the interaction of atmospheric air and precipitation with the rocks of the dumps, the oxidation-reduction medium changes and, as a result, very different associations of minerals are formed. The gas/aerosol ratio determines the amount of material leached from the dumps. The more "dry" the gas, the less elements are released into the environment in terms of quantity and variety.
The mineral composition of the newly formed crusts in fluid conductors can be used to infer the initial physico-chemical conditions of the interaction of atmospheric air and water with the rocks of the dumps.
5. Conclusion
The received information has shown a significant gaseous transport of trace elements. A wide spectrum of elements was released from burning coal or hot slag, not only volatile anion-forming As and Sb but also elements that are traditionally considered as refractory (Ti, Fe).
The snow cover pollution in the region of Novosibirsk CHP-2 and CHP-3 (used the Kuzbass coal) and the gas condensates composition produced by experimental burning of Kuzbass coal were compared. A group of major elements (Ti, Fe, Ga, V, Ni) has identical concentrations in the dissolved part of the snow cover and in the condensate. These are non-volatile and inactive elements that accumulate in solution. The alkali metals and arsenic (Na, Mg, K, Ca, Cu, Zn, As) with a higher migratory capacity have a higher concentration in gas condensates.
A number of mobility elements in the system air / snow is Ti <Fe <Ga <V <Ni <Sr <Rb <Mn <Ca <Pb <Cd <Cu <K <Zn <Cr <Na <Mg <As.
Thermodynamical modeling shows that depends on the initial temperature, the interaction of atmospheric air and the precipitation rocks of the dumps, the oxidation-reduction medium changes and as a result, very different associations of minerals are formed. The ratio of gas / aerosol determines the amount of material leached from the rock dumps. The more "dry" the gas, the less elements are leached into the environment in terms of quantity and variety.
High concentrations of trace elements in the condensates collected on the burning slag heaps as well as our laboratory experiments have shown that gaseous transport of a wide spectrum of elements can take place in high-temperature anthropogenic processes. The problem of element gaseous transport in anthropogenic processes should attract much more attention than what it receives now, because emissions of toxic trace elements can potentially occur in large scale (e.g. subterranean combustion of spoil tips or forest fires) having serious environmental impact.